Plug-in hybrid electric vehicles (PHEVs) have generated significant interest for their potential to decrease dependence on imported oil and to cut pollution and greenhouse gas emissions. While hybrid electric vehicles (HEVs) rely on their internal combustion engines to recharge their batteries, PHEVs generally have larger batteries and can be recharged by plugging into an outside electricity source, such as a standard home outlet (Figure 1). As a result, PHEVs are potentially more efficient and cleaner than HEVs, in part because more of their energy can come from clean, renewable sources.
A critical consideration in PHEV development is how energy is produced and used. More flexible and intelligent PHEV energy management strategies can save energy and produce lower emissions. This can translate to an increase in fuel economy of 5 to 10 more miles per gallon of gas for a typical PHEV that already gets 60 miles per gallon. We discuss one strategy to optimize energy management by accounting for vehicle position, speed and acceleration, trip progress, roadway characteristics, traffic conditions, and battery recharging opportunities at intermediate stops. We then evaluate this energy management strategy using an example trip, and find that it can result in substantial efficiency gains.
Energy Management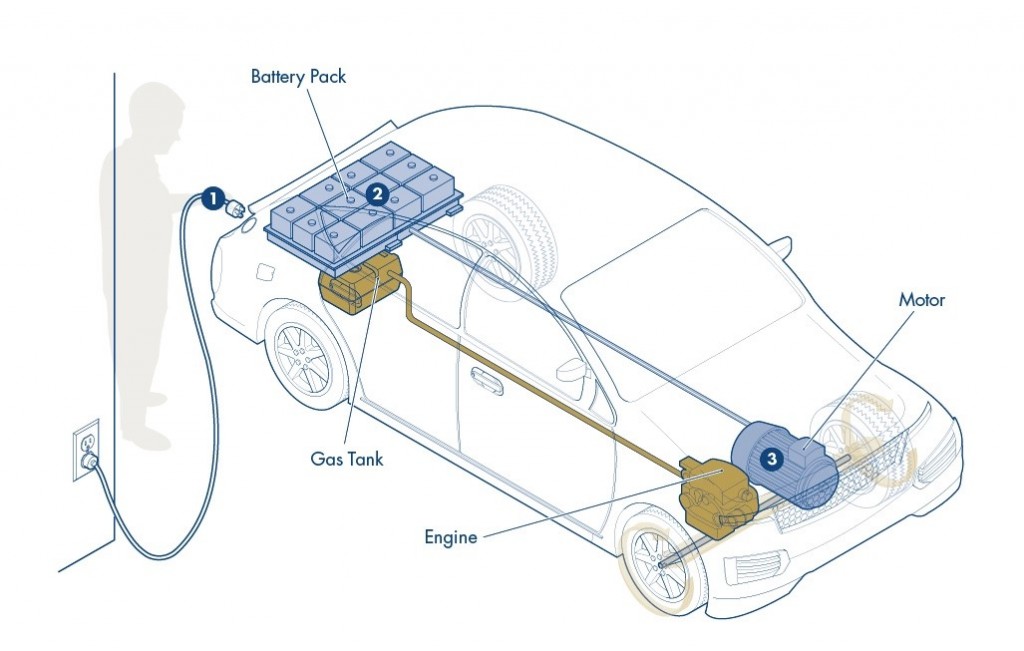
Energy management in PHEVs depends on factors such as the power required to move the vehicle forward and the battery’s state of charge (SOC). SOC is the equivalent of a fuel gauge for the battery pack, ranging from 0 percent (empty) to 100 percent (full). The battery in a PHEV operates best at moderate levels of SOC and is less efficient at very low or very high levels of SOC. Therefore, PHEV energy management strategies tend not to leave the battery pack empty or charge it fully in order to help preserve the battery life.
An important aspect of PHEV energy management is controlling how the battery charge is used and depleted. The charge is expected to drop to a low level when the vehicle reaches a destination where it can be plugged in and recharged. A battery reaching its lowest allowable level means that the vehicle has maximized its use of electricity drawn from the grid, which is usually a cheaper and cleaner energy source than fossil fuels.
Batteries should be used in a way that optimizes energy efficiency for a particular trip. One commonly used strategy is to run the PHEV solely on electric energy until its battery reaches a certain charge level, and then switch to gasoline for the rest of the trip. This strategy—referred to as the binary mode—may result in gasoline consumption where battery use is desirable (such as in stop-and-go traffic or while going down a steep hill). Therefore, the binary mode may not minimize fuel consumption and emissions for a trip.
To develop an intelligent energy management strategy for PHEVs, we first estimated the amount of power the vehicle needs on a continuous basis for the duration of an example trip. Vehicle power is generally a function of vehicle speed, acceleration, mass, and road grade. Therefore, second-by-second vehicle speed profiles must be synthesized based on roadway and traffic information. Fortunately, such real-time traffic information has become increasingly available in the past several years. California’s Freeway Performance Measurement System (PeMS), for instance, provides traffic measurement data every five minutes from thousands of loop detectors on the state’s freeway system.
Using PeMS data, we calculated a detailed profile of the example trip before it began. Average traffic speed measurements and a large database of real-world vehicle speed profiles allowed us to estimate second-by-second vehicle speeds and corresponding accelerations. We then calculated the vehicle’s location at each second along the trip and obtained road grade values for these locations. Using this speed, acceleration, and road grade information, we estimated second-by-second vehicle power requirements for the trip.
The intelligent energy management strategy yields fuel savings between 10 and 12 percent.
We used the estimated vehicle power requirements along with PHEV powertrain models to determine an optimal strategy for depleting the battery charge for the trip. This was done by estimating the engine’s power supply and the associated drop or gain in the battery SOC at each second based on the commute variables (elevation, distance, etc.). This allows us to determine a trip’s optimal charge-depleting profile that uses minimal power from the internal combustion engine while ensuring that 1) vehicle power requirements are met for the entire trip, and 2) the battery SOC never drops below a pre-set threshold. Solutions may also incorporate battery-recharging opportunities at intermediate stops along a trip chain.
Evaluation and Results
To evaluate the effectiveness of our intelligent energy management strategy, we modeled a commute trip of about 25 miles each way along Interstate 210 in Los Angeles and San Bernardino Counties based on real-world traffic patterns. We assumed a westbound morning commute beginning at 8 am, and an eastbound afternoon commute beginning at 4 pm on the same day. During the morning commute, the PHEV would experience heavy congestion in the first half of the trip, and then no congestion thereafter. During the afternoon commute, the PHEV would experience moderate congestion throughout the trip.
We set the range of allowable states of charge between 20 and 80 percent of capacity in order to preserve the battery life. To evaluate the intelligent energy management strategy at the individual trip level, we assumed that the starting SOC is at the maximum allowable charge, 80 percent. Figures 2 and 3 compare SOC profiles of our intelligent energy management strategy and the binary mode strategy, which is commonly used in current PHEVs. Unlike the binary mode, the intelligent energy management strategy SOC reaches the minimum charge level at the end of each trip because the strategy uses roadway and traffic information to manage the hybrid powertrain. In some instances, especially during the westbound trip, our intelligent energy management strategy increases the SOC significantly as it takes full advantage of regenerative braking opportunities during traffic congestion and while traveling downhill.
The previous example assumes a full charge at the workplace, but what if we can only partially charge at the workplace? If the two trips are considered as one trip chain, and we know ahead of time that we can only partially recharge the battery at the workplace, our intelligent energy management strategy can still optimize energy consumption for the entire trip chain. Figure 4 compares SOC profiles of the commute trip chain that includes 30 percent SOC gain from a limited battery recharging opportunity at the workplace. In the binary mode strategy, the PHEV consumes the electricity very quickly, and the SOC reaches the minimum limit far before the individual trips end. After the SOC reaches its minimum limit, the PHEV uses only fuel. In contrast, the intelligent energy management strategy uses the electricity as appropriate, such as to supplement the internal combustion engine when going up hills, which allows the SOC to reach the minimum limit at the end of the trip chain.
The example trips show that our intelligent energy management strategy can save 14 percent of fuel consumed on the westbound trip and 9 percent on the eastbound trip compared to the binary mode strategy (Figure 5). Figure 6 estimates fuel consumption if the two commute trips are combined into a single trip chain with one intermediate stop at the workplace. Compared to the binary mode strategy, the intelligent energy management strategy yields fuel savings between 10 percent (no battery recharging at work) and 12 percent (fully recharging at work).
Conclusion
Recent advances in PHEV technologies can greatly improve fuel efficiency and reduce harmful vehicle emissions. But most PHEVs use a binary-mode energy management strategy, which does not adapt to real-time driving situations or use each energy source as efficiently as possible.
In this research, we propose an intelligent energy management strategy for PHEVs that takes into account real-time information of vehicle position, roadway characteristics, traffic conditions, and if applicable, battery-recharging opportunities at intermediate stops in a trip chain to optimize fuel efficiency. Our calculation for an example commute trip shows that the intelligent energy management strategy can reduce fuel consumption by between 9 and 14 percent. This corresponds to a fuel efficiency increase from 60 to between 65 and 70 miles per gallon of gasoline equivalent.
Plug-in hybrid electric vehicles in the current market are already more fuel-efficient than conventional gasoline or diesel vehicles. By utilizing real-time information, PHEVs can save even more fuel for drivers and further reduce emissions.
This article is adapted from “Development and Evaluation of an Intelligent Energy-Management Strategy for Plug-in Hybrid Electric Vehicles,” originally published in the IEEE Transactions on Intelligent Transportation Systems.
Further Readings
Electrification Coalition. 2013. State of the Plug-in Electric Vehicle Market, Washington DC.